Electrolysis of water provides a sustainable pathway toward storing electrical energy from renewable sources in the form of chemical bonds. Among the two half-cell reactions involved in water splitting, the anodic oxygen evolution reaction (OER) requires electric potentials significantly higher than the thermodynamic limit to achieve desirable electrochemical rates, making it a prime source of inefficiency. Precious metal-based oxides, such as IrOx, have been largely considered as standard electrocatalysts for OER in acidic electrolytes. On the other hand, alkaline water electrolyzers allow for a wider range of non-precious metal-based electrocatalysts (i.e., earth abundant 3d transition metal (TM) oxides) for OER due to their relatively higher stability under these conditions. Among these, non-stoichiometric mixed ionic and electronic conducting oxides belonging to the perovskite family of the general form An+1BnO3n+1 (n=1, 2, 3âŚ; A and B represent alkaline earth/rare earth metal and transition metal (TM) cations, respectively) have been explored. Generally, the bulk electronic structure of the B-site cations in these oxides has been correlated to their electrocatalytic activity for OER under the assumption that the initial state of the oxide surface remains mainly unperturbed during OER.
We have recently shown that structural changes occur at the interface of non-stoichiometric mixed metal oxides with a liquid alkaline electrolyte under oxidative OER potentials. These changes lead to the in-situ generation of an OER active amorphous 3d transition metal oxyhydroxide shell on top of the parent oxide core. This is generally induced by the dissolution of the A-site alkaline earth metal cations in the electrolyte. We find that the extent of A-site alkaline earth metal cation dissolution from the oxide is linked to: (i) the global crystal symmetry of the oxide with n=1 R-P phases exhibiting higher rates of dissolution as opposed to n= perovskites; (ii) the oxide reducibility, which correlates with the strength of metal-oxygen bonds; and (iii) the lattice strain induced by the differences in the ionic radii of the rare earth and alkaline earth metal cations in mixed A-site compositions. The extent of B-site transition metal cation dissolution is significantly lower, with highly oxophilic metal cations, such as Fe and Mn, exhibiting higher dissolution than Co and Ni. These findings demonstrate that mixed metal oxides evolve under OER conditions, advocating for the need to consider the changing nature of their surface structure at electrochemical solid-liquid interfaces when developing design principles to optimize their performance.
Biography
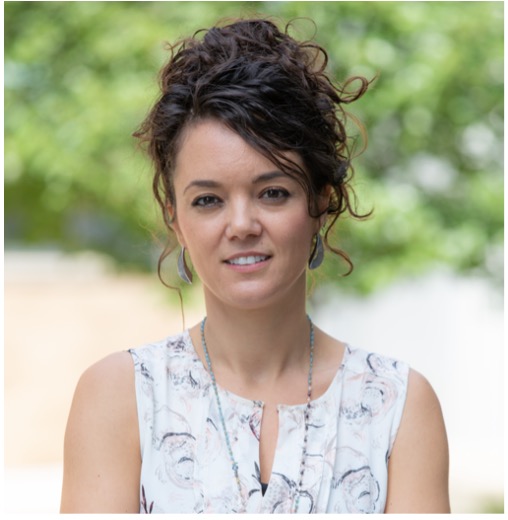
Eranda Nikolla is a Professor of Chemical Engineering at the University of Michigan-Ann Arbor, MI. Prior to this, she was a Professor of Chemical Engineering and Materials Science at Wayne State University, Detroit, MI. Her research interests focus on the development of heterogeneous catalysts and electrocatalysts for chemical conversion processes and electrochemical systems (i.e., fuel cells, electrolyzers). As an integral part of engineering catalytic/electrocatalytic structures, Nikolla has implemented a paradigm which involves a combination of controlled synthesis, advanced characterization, kinetic measurements, and quantum chemical calculations to unearth the underlying mechanism that governs their catalytic performance for targeted reactions. Her groupâs impact to catalytic science has been recognized through the National Science Foundation CAREER Award, the Department of Energy Early Career Research Award, Camille Dreyfus Teacher-Scholar Award, the Young Scientist Award from the International Congress on Catalysis, the 2019 ACS Women Chemists Committee (WCC) Rising Star Award, the 2021 Michigan Catalysis Society Parravano Award for Excellence in Catalysis Research and Development, and the 2022 ACS Catalysis Lectureship for the Advancement of Catalytic Science.