Ammonia (NH3) synthesis is a key industrial process, being of vital importance for the production of fertiliser, explosives, and fine chemicals. Traditional promoted Fe Haber-Bosch catalysts require high temperatures and pressures to operate, at great economic and environmental expense due to the significant energy consumption required for NH3 synthesis. Hence, there is interest in developing novel NH3 synthesis catalysts that are highly active and can operate efficiently under much less extreme conditions. Ternary and quaternary metal nitride materials have been identified as promising candidates, with the η-carbide structured Co3Mo3N found to be even more active than the industrially well-established promoted Fe catalysts. Experimental studies have revealed that lattice N in Co3Mo3N is highly active, with significant loss of N occurring under elevated temperatures, yielding the Co6Mo6N phase; the Co3Mo3N phase is regenerated upon the introduction of N2[1]. Complementary computational studies revealed that the activity of lattice N enables an associative Mars van Krevelen type mechanism, with surface N vacancies serving as active sites for N2 adsorption and activation; the novel associative Mars van Krevelen mechanism was also found to be less energetically demanding than the conventional dissociative Langmuir-Hinshelwood that has been well-established for the traditional promoted Fe catalyst[2].
The isostructural Fe3Mo3N catalyst has also been identified as highly active for NH3 synthesis, although unlike Co3Mo3N, no significant loss of lattice N was observed, implying that if any loss of lattice N does occur, it is confined only to surface lattice N[3]. Hence, there is interest in investigating the energetics of N vacancy formation for Fe3Mo3N, and determining their precise role in the mechanism for NH3 synthesis over this catalyst, in order to compare with the Co3MoN system and identify similarities and differences between these closely related systems. Furthermore, a complete understanding of each of the Co3Mo3N and Fe3Mo3N systems is a perquisite for future studies investigating the quaternary Co/Fe molybdenum nitride system. In the present work, plane-wave density functional theory techniques are applied to investigate surface N vacancy formation in Fe3Mo3N, and both the dissociative Langmuir-Hinshelwood and associative Mars van Krevelen mechanisms that have been explored in detail for Co3Mo3N. The calculations reveal which processes represent kinetic bottlenecks for ammonia synthesis, and will allow for a detailed comparison with the Co3Mo3N system, providing new insights into the catalytic behaviour of ternary metal nitride systems.
[1] Hargreaves, J. S. Applied Petrochemical Research, 2014, 4, 3.
[2] Zeinalipour-Yazdi, C. D.; Hargreaves, J. S. J.; Catlow, C. R. A. J. Phys. Chem. C, 2018, 11, 6078.
[3] Hunter, S. M. Molybdenum nitrides: Structural and reactivity studies, PhD Thesis, University of Glasgow, 2012
Click on the link below to view a recording of the presentation:
Biography
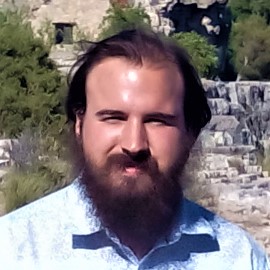
Michael Higham completed a PhD in Chemical Science and Technology at the Institut Català d’Investigació Química (ICIQ, Catalan Institute of Chemical Research) in November 2017. Michael joined Prof. Sir Richard Catlow’s group at Cardiff University in August 2018, supported by the GCRF (Global Challenges Research Fund) START (Synchotron Techniques for African Research and Technology) project, providing computational insights to support experimental work utilising the synchrotron facilities at Diamond, focusing on Cu-based catalysts for methanol synthesis from CO2. In September 2021, Michael joined Prof. Catlow’s UCL team as a postdoctoral researcher and is currently investigating metal nitride catalysts and nitrogen looping materials for ammonia synthesis, in collaboration with Prof. Justin Hargreaves at the University of Glasgow.